The Greening of Hydrogen
The Hydrogen Metals Standard
26 November 2021
The greening of hydrogen
COP26 – The background
Delayed by one year due to the pandemic, the 26th Conference of the Parties to the United Nations Framework Convention on Climate Change (COP26) in Glasgow, Scotland, in November 2021 is the scheduled occasion for the nations of the world to review their commitments to control global warming that they made in Paris at the end of COP21 in 2015.
The Paris Agreement is a commitment to take actions to limit the increase in global temperature compared to pre-industrial levels to less than 2.0oC and aim for a limit of 1.5oC. It is generally accepted that to achieve the lower limit, world emissions of carbon need to halve over the decade to 2030 and by 2050 reach net zero – a state in which the amount of carbon entering the environment is no greater than the amount absorbed by it. Actions towards this goal of decarbonisation encompass phasing out the use of coal and natural gas, electrification of transport, controlling deforestation and making agriculture more sustainable.
A total of 194 countries have submitted Nationally Determined Contributions (NDCs) or climate action plans to the United Nations, and as at July 2021 the total of countries adopting a net-zero target accounted for 80% of world GDP. Carbon reduction targets are partly determined by legacy issues: a nation’s degree of dependence on fossil fuels or the extent of its economic development. Accordingly, some NDCs – notably from Saudi Arabia, Australia, China and India – do not fully meet the Paris targets. Another issue for debate is the provision of funds from advanced economies to support the climate control and mitigation efforts of developing nations, which has not matched expectations.
Although momentum towards global decarbonisation has developed, there is uncertainty (which will continue beyond COP26) about how much can be achieved. On the eve of the Glasgow conference, the United Nations Environment Programme (UNEP) published its 2021 Emissions Gap Report, in which it ominously declared that the updated NDCs still fail to put the world on track to meet the Paris Agreement’s global warming target and that “unless there are immediate, rapid and large-scale reductions in GHG emissions, limiting warming to 1.5oC or even 2oC by the end of the century will be beyond reach”. Even if targets are tightened, other barriers loom: an increase in the supply of the necessary raw material resources – nickel, cobalt and lithium, to name a few – is a massive challenge to meet in the time available.
Decarbonisation and hydrogen
A Green Energy Transition is needed to replace fossil-derived energy (coal, oil and natural gas) with energy from renewable sources (wind and solar). In 2020, according to the International Energy Agency in its latest World Energy Outlook, total world CO2 emissions were 34.2 gigatonnes (Gt). The energy sector (electricity, heat and other forms of energy) was the largest emitter of carbon with around 44% of the world total. Here, reductions in emissions can be achieved by transitioning from fossil fuels to renewable sources, along with more efficient energy use. Industry, with 26% of CO2 emissions, can also incorporate renewable energy-based systems. Transport, which was responsible for 21%, can reduce emissions by using a range of electrified technologies and alternative fuels. In each of these sectors, hydrogen derived from renewable sources is expected to be an increasingly important part of the energy transition.
CO2 emissions by sector
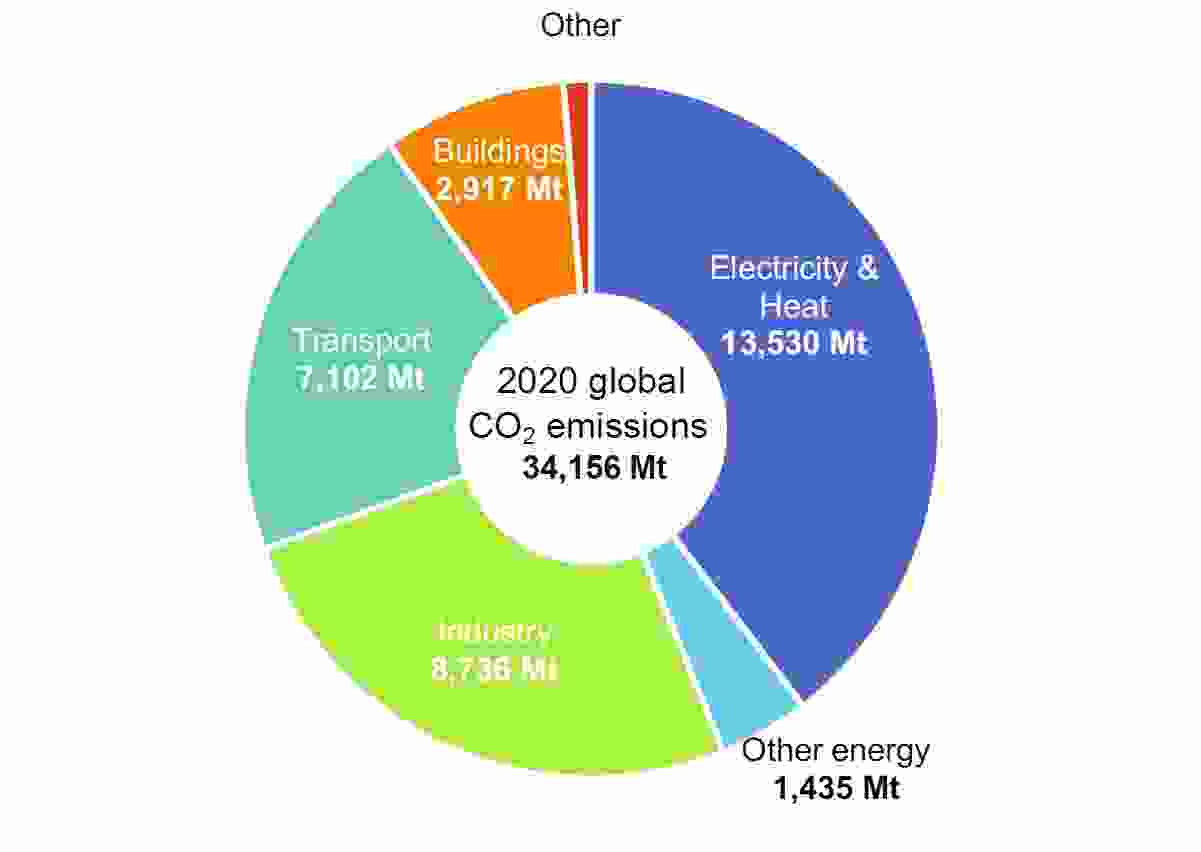
Source: IEA, World Energy Outlook October 2021
Hydrogen has very high energy density per unit of weight – 2.6 times that of natural gas; when burned, it has no carbon emissions that would contribute to global warming or air pollution, and it is a stable vector for energy storage and can be easily transported in gas pipelines. On the other hand, being the lightest of the elements, hydrogen has poor energy density by volume, so it has to be compressed or liquefied for transport or use; and, as it does not occur naturally, must be derived from other molecules such as natural gas or water. This incurs a loss of efficiency in the process, making the cost of producing the hydrogen always higher than the cost of the input power, with implications for the competitiveness of hydrogen in applications where renewable electricity can be used directly, as in batteries for electric vehicles or in heat pumps for domestic heating.
Hydrogen pros and cons
Pro
-
High density by weight
-
No carbon emissions when combusted or converted to electricity
-
Vector for energy storage
Con
-
Does not occur naturally so costs energy to produce
-
Low density by volume, must be compressed or liquefied for use
Hydrogen will therefore likely make its contribution to decarbonisation in energy storage and in applications which are energy-intensive, where alternatives are unsatisfactory or where emissions are difficult to abate. In industry, it is already important for production of ammonia and other chemical products. Hydrogen would decarbonise these processes if sourced from renewable electricity instead of from natural gas. In steelmaking, combining hydrogen with coke in a blast furnace as a source of heat can make marginal reductions in carbon emissions, but alternatively, hydrogen can replace coke as the reducing agent in a direct reduction-electric arc furnace, resulting in close-to-zero carbon outputs. In Europe, 14 such projects are planned, nine of which have specific production targets amounting to 13% of annual EU steel production (source: https://bellona.org).
As an energy vector, hydrogen can be blended with natural gas to generate electricity or for combustion in domestic boilers, but it will have a far greater effect on decarbonisation as a flexible store of energy to secure constant electricity supply. Increasing dependence on electricity generated by intermittent solar and wind power brings the challenge of balancing electricity flow and providing reserves for whenever supply diminishes. Hydrogen produced and stored when surplus power is available is ideally positioned to meet this need.
In the transport sector, combining hydrogen with oxygen in a fuel cell to produce electricity, with only water as a by-product, is the main alternative to batteries for the electrification of vehicles, particularly in heavy-duty, long-range transport applications in which the weight and limited range of batteries would not satisfy the operating requirements. Long-distance trucks, trains, some ships and even aircraft, if a suitable degree of compression or liquefaction of hydrogen can be achieved, are all potential adopters of fuel cells. Internal combustion engines running on 100% hydrogen instead of diesel are also being developed for use in the heavy-duty sector.
The production of hydrogen
The routes to hydrogen, broadly in order of environmental credentials and carbon footprint are:
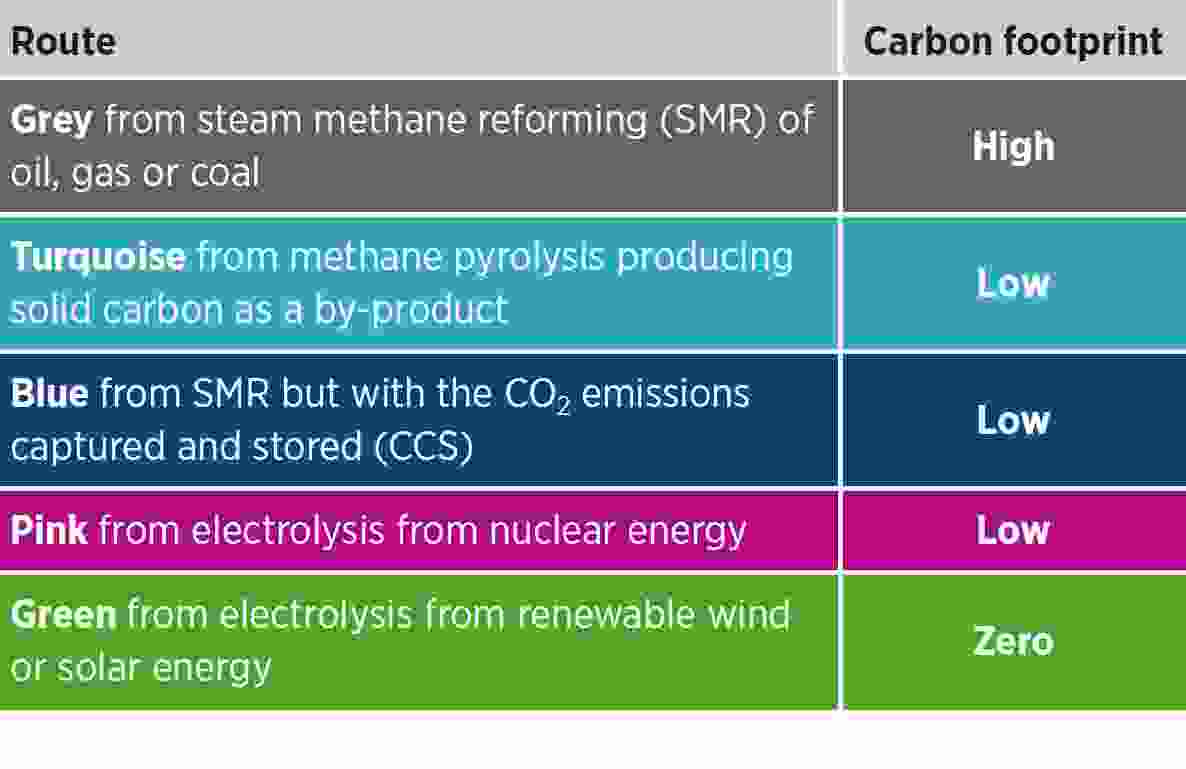
Source: SFA (Oxford)
At present, 95% of hydrogen is made from hydrocarbons. This ‘grey’ hydrogen is cheap to produce, as low as $1 per kilogram (depending on the price of natural gas), but the associated greenhouse gas emissions are high. ‘Blue’ hydrogen, also from fossil fuels but with the carbon emissions captured and sequestered, costs a little more but may be only an interim solution: the process captures only 85-95% of emissions while the long-term liabilities of storing large volumes of CO2 in underground geological or ex-industrial spaces are complex and hard to quantify. ‘Pink’ (sometimes named ‘yellow’) hydrogen, using nuclear energy as a source of electricity, is low-carbon but expensive and far from being a popular generating option. The only truly acceptable zero or close-to-zero emissions route is ‘green’ hydrogen made by using the infinitely renewable electricity from solar panels or wind turbines in an electrolyser to split water into hydrogen and oxygen.
The colours of hydrogen
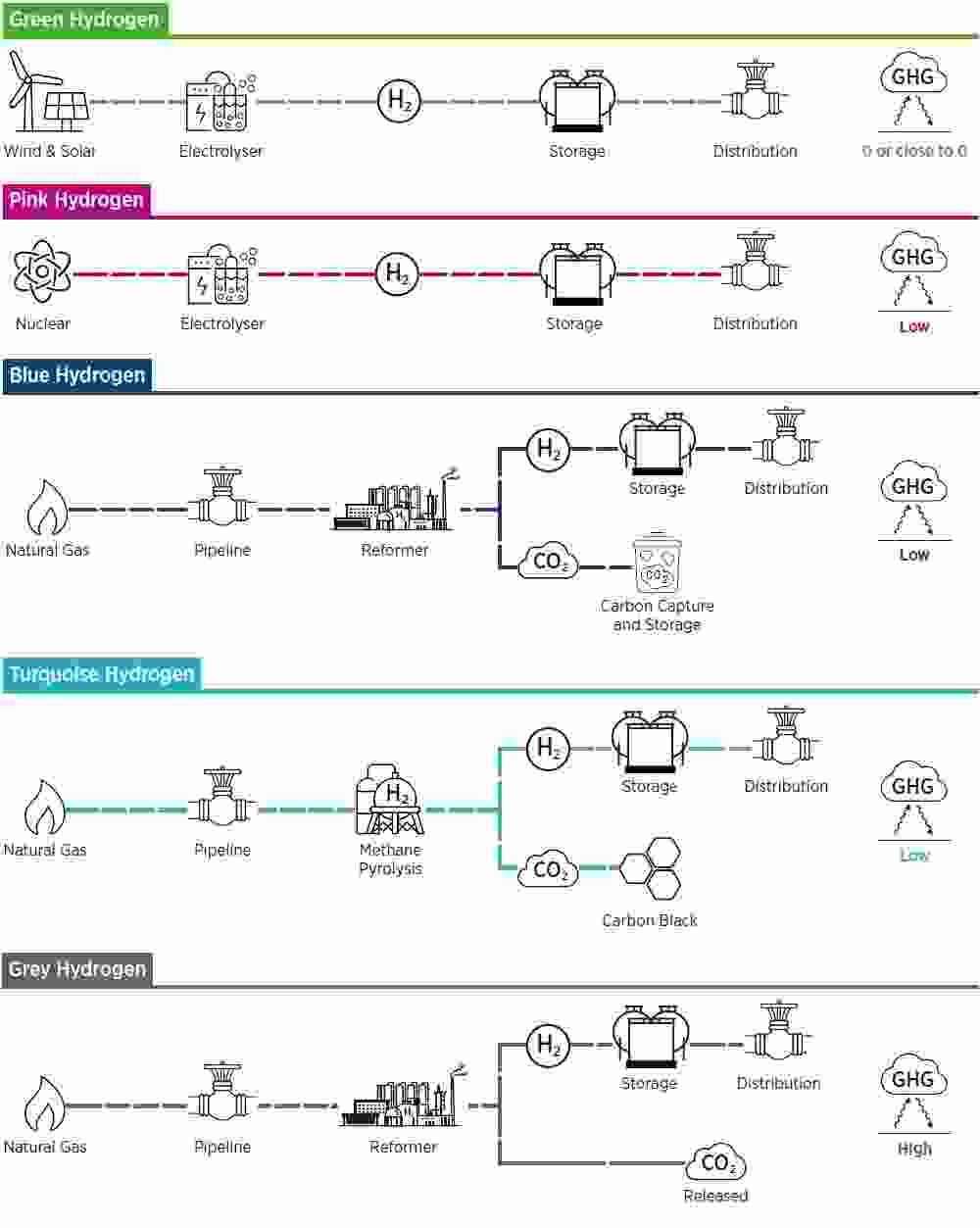
Source: Energy Industry Review
Hydrogen and electrolysers have accordingly become a focus of technology development. The EU’s Hydrogen Strategy puts green hydrogen in the spotlight as a priority to reach carbon neutrality by 2050 and for the global effort to implement the Paris Agreement while working towards zero pollution. In its 2020 Green Deal, the EU targeted by 2030 an electrolyser capacity of 40 GW within its borders and another 40 GW in nearby countries that can export hydrogen. The United Kingdom is aiming for 5 GW of low carbon hydrogen capacity by the same date.
Announced clean hydrogen capacity through 2030
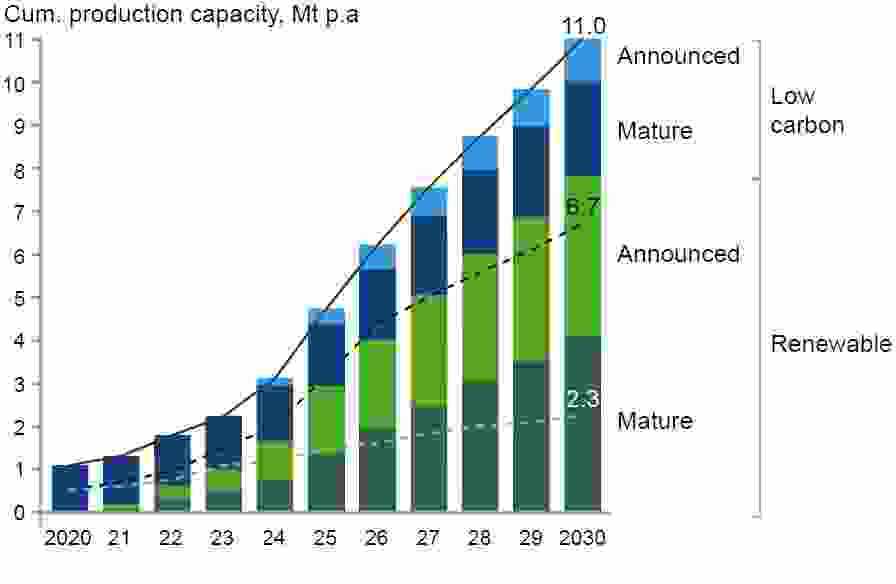
Source: Source: Hydrogen Council, McKinsey & Company, Hydrogen Insights July 2021 Update
The cost of producing green hydrogen – between $2.5 and $6 per kg at present – must reduce substantially for it to become a serious contender, yet given adequate investment and sufficient supply of materials this should be achievable in the long term. The cost of generating the renewable electricity on which green hydrogen relies has declined over time to the point where the levelised cost of electricity (LCOE – the discounted lifetime cost of building and operating a generating plant) from solar and wind is already below the cost of electricity derived from coal and natural gas. Current world electrolyser capacity is about 3 GW; scaling this up to 70 GW by 2030, as predicted by McKinsey & Company in a July 2021 Hydrogen Insights report for the Hydrogen Council, will go a long way to reduce electrolyser CAPEX.
LCOE of energy from fossil and renewable sources
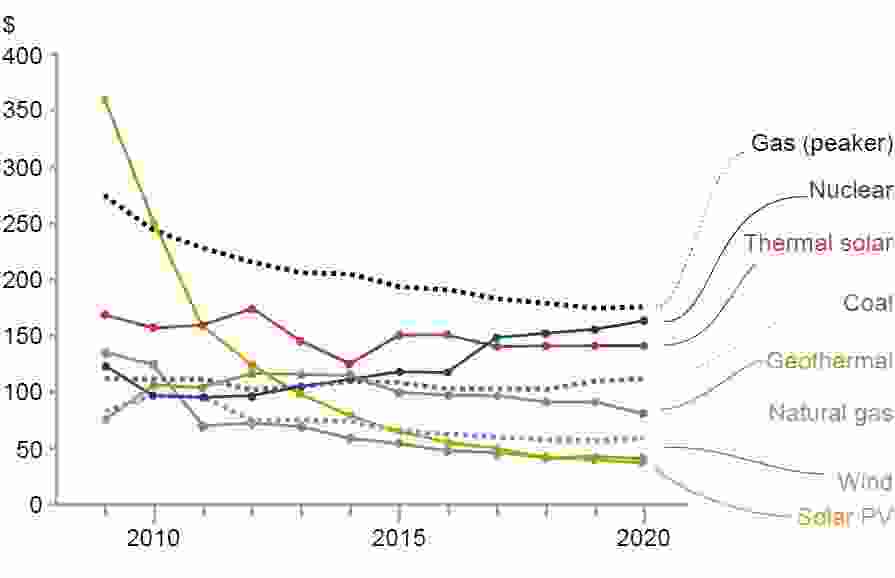
Source: RCraig09 – Own work, CC BY-SA 4.0, https://commons.wikimedia.org/w/index.php?curid=99427431
As a result of these trends, the cost of green hydrogen is likely to fall to levels competitive with existing methods of production. According to BloombergNEF, the price of green hydrogen using proton exchange membrane (PEM) electrolysis could be comparable with blue hydrogen by 2030. Credits for renewable electricity production and subsidies for electrolysers, such as those currently in force in the US, could even enable green hydrogen to compete with grey in a few years’ time, according to Morgan Stanley.
Carbon pricing is recognised as a potentially pivotal element in steering towards net zero. The cost of carbon credits as indicated by the IHS Markit global carbon index in the following chart is trending upwards (80% of the constituent data is from the markets for carbon credit futures in the EU27 and California). A more universal adoption of carbon pricing, whether as an Emissions Trading System (ETS) or as a carbon tax, would reinforce the future competitiveness of green hydrogen as it would factor in the real cost of producing grey and blue hydrogen.
Global carbon index (USD)
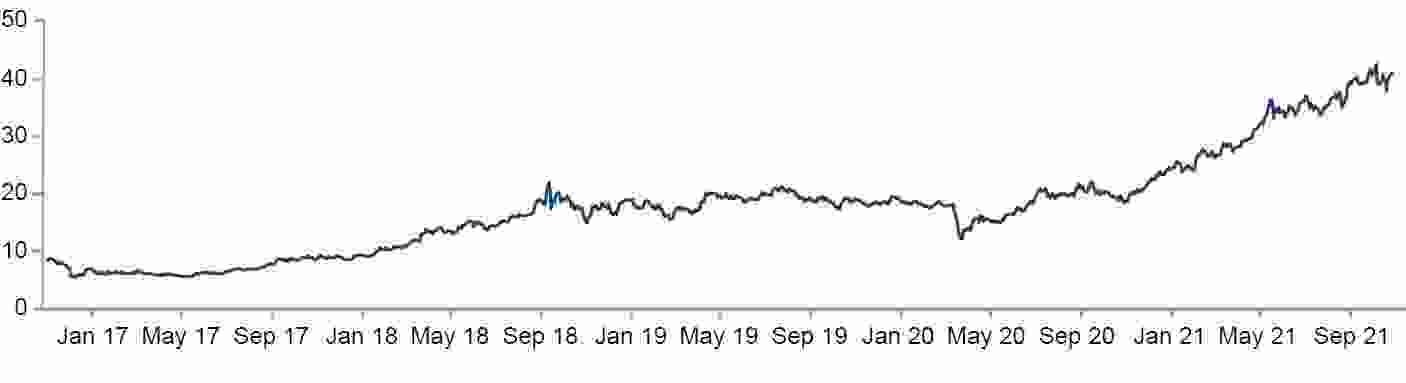
Source: IHS Markit
Electrolyser options
There are four types of water electrolyser for producing green hydrogen. Alkaline (ALK) and PEM electrolysers are already in commercial use at scale. Both are considered expensive relative to the current cost of producing grey hydrogen. Anion exchange membrane (AEM) and solid oxide (SOE) electrolysers are still under development, although they may hold great potential. All four technologies have their challenges, ranging from use of critical materials to performance, durability and maturity. There is no outright winner, and future use will depend on the application to which each is put.
Current (2020) state of the art for PEM and ALK technologies
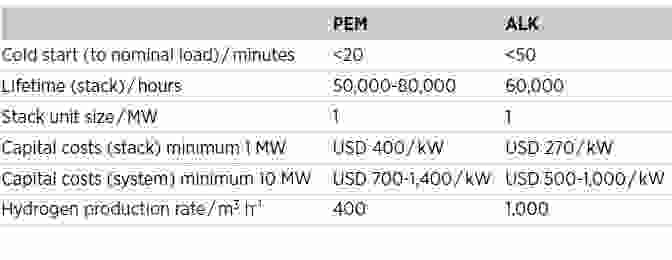
Source: (1) IRENA (2020), Green Hydrogen Cost Reduction: Scaling up Electrolysers to Meet the 1.5⁰C Climate Goal, International Renewable Energy Agency, Abu Dhabi. (2) Yujing Guo et al (2019), IOP Conf. Ser.: Earth Environ. Sci., 371 042022
ALK electrolysers have a simple design and manufacturing process; they benefit from high efficiency and a large scale and are relatively low-cost, and they run best on a base load without significant fluctuations. PEM electrolysers rely on platinum-group metal catalysts, so at present are 50-60% more expensive than ALK, but they demonstrate good dynamic response and the capability to work well under intermittent operation, a feature of renewable electricity supplies. The focus for development of ALK electrolysers is to improve their dynamic response and capability for intermittent operation, while for PEM it is to operate at larger scale with higher efficiency and lower cost.
AEM’s potential combines the less harsh operating environment of ALK with the simplicity and efficiency of PEM but without the expensive metals, but overall performance and stability is lacking. SOE’s potential lies in its high temperature efficiency, so it can use cheap metals, but its main challenge is durability.
Electrolyser technologies compared
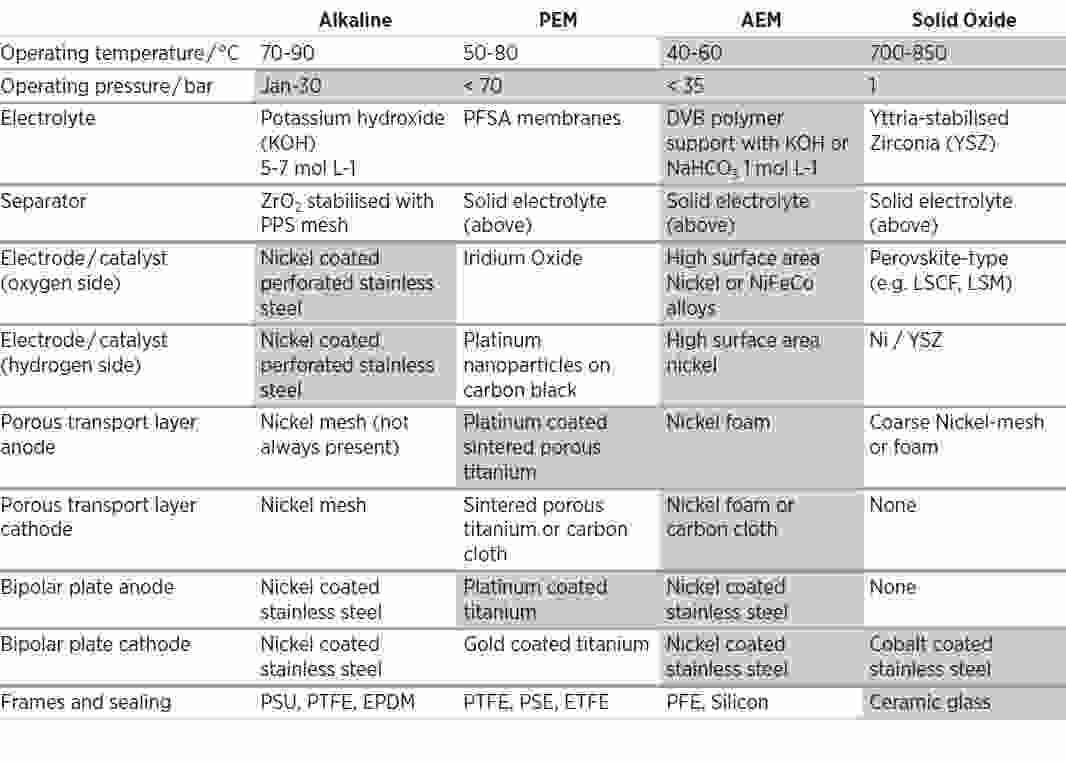
Source: IRENA
Hydrogen and platinum-group metals (PGMs)
Deep links exist between hydrogen and PGMs in the fields of energy, industry and transport, and demand for PGMs in these applications will expand progressively over the next decades, with the strongest growth coming from the greening of hydrogen production using electrolysers.
In PEM electrolysers, the electrode at which hydrogen evolves (the cathode) uses platinum-based materials and the electrode at which oxygen is generated (the anode) is usually based on iridium.
At the core of the PEM electrolyser, the catalyst-coated membrane (CCM) containing the PGMs represents about 10% of the entire system cost. Reducing this cost element will involve reduction of PGM loadings, particularly iridium which is a by-product of platinum refining and which therefore has limited availability.
PEM electrolyser cost breakdown
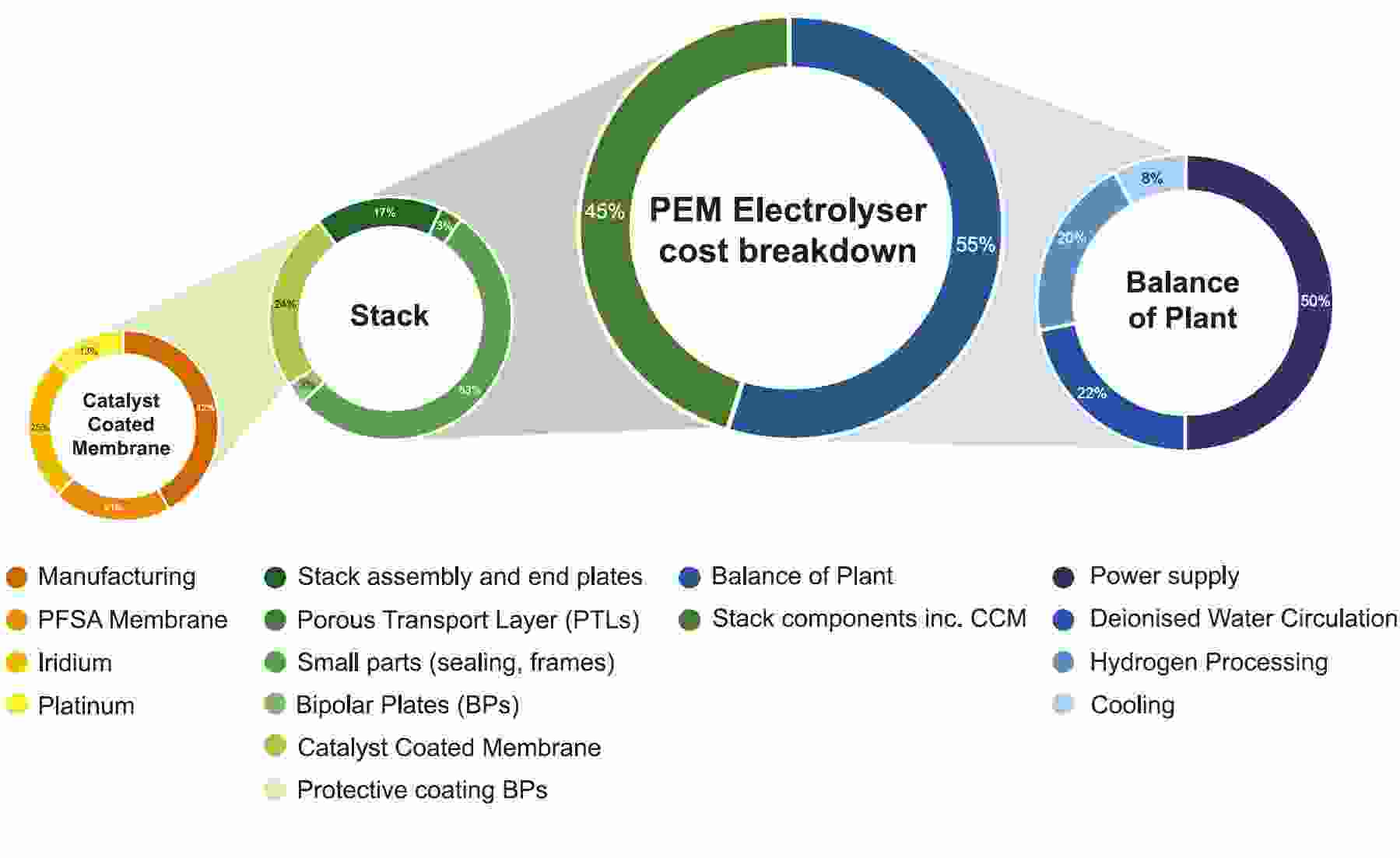
Source: IRENA
Iridium and platinum would be hard to substitute entirely as they are technically the best fit for PEM electrolysers. Innovation to reduce the intensity of PGM use is based on some familiar themes in catalyst surface chemistry and engineering – for example, by increasing the catalyst surface area and using thinner layers of catalyst coating material. PGM fabricators have had high success rates using these methods over many years in reducing PGM loadings while at the same time increasing the performance of their products – autocatalysts and fuel cells being prime examples.
In PEM fuel cells, whether used for transport or industrial applications including back-up, portable or grid-independent power, platinum is used as the catalyst at both the cathode and anode, with ruthenium added to protect the platinum from impurities in the hydrogen feed. Green hydrogen is higher purity than grey, and as uncontaminated hydrogen becomes more widely available there will be scope to reduce the ruthenium content.
Hydrogen engines may not require PGM autocatalysts as gasoline and diesel engines do, because of the absence of hydrocarbon and CO emissions. There may be some NOx emissions, but they can be limited by controlling engine temperature. Removal of any residual emissions can be performed by selective catalytic reduction (SCR), which does not use PGMs, although there might – as in diesel catalyst NOx removal systems – be a little platinum used to remove any ammonia slip from the SCR. However, platinum and iridium demand could gain from previously diesel engines now requiring spark plugs.
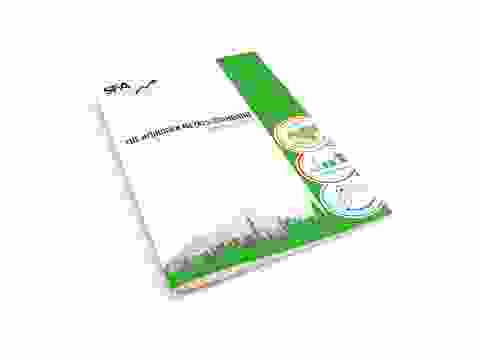
Disclaimer, copyright & intellectual property
SFA (Oxford) Limited has made all reasonable efforts to ensure that the sources of the information provided in this document are reliable and the data reproduced are accurate at the time of writing. The analysis and opinions set out in the document constitute a judgement as of the date of the document and are subject to change without notice. Therefore, SFA cannot warrant the accuracy and completeness of the data and analysis contained in this document. SFA cannot be held responsible for any inadvertent and occasional error or lack of accuracy or correctness. SFA accepts no liability for any direct, special, indirect or consequential losses or damages, or any other losses or damages of whatsoever kind, resulting from whatever cause through the use of or reliance on any information contained in the report. The material contained herewith has no regard to the specific investment objectives, financial situation or particular need of any specific recipient or organisation. It is not to be construed as a solicitation or an offer to buy or sell any commodities, securities or related financial instruments. The recipient acknowledges that SFA is not authorised by the Financial Conduct Authority to give investment advice. The report is not to be construed as advice to the recipient or any other person as to the merits of entering into any particular investment. In taking any decision as to whether or not to make investments, the recipient and/or any other person must have regard to all sources of information available to him. This report is being supplied to the recipient only, on the basis that the recipient is reasonably believed to be such a person as is described in Article 19 (Investment professionals) or Article 49 (High net worth companies, unincorporated associations etc.) of the Financial Services and Markets Act 2000 (Financial Promotion) Order 2005.
© Copyright reserved. All copyright and other intellectual property rights in any and all reports produced from time to time remain the property of SFA and no person other than SFA shall be entitled to register any intellectual property rights in any report or claim any such right in the reports or the information or data on the basis of which such reports are produced. No part of any report may be reproduced or distributed in any manner without written permission of SFA. SFA specifically prohibits the redistribution of this document, via the internet or otherwise, to non-professional or private investors and accepts no liability whatsoever for the actions of third parties in reliance on this report.
Brought to you by
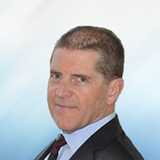
Jeremy Coombes
Independent Consultant
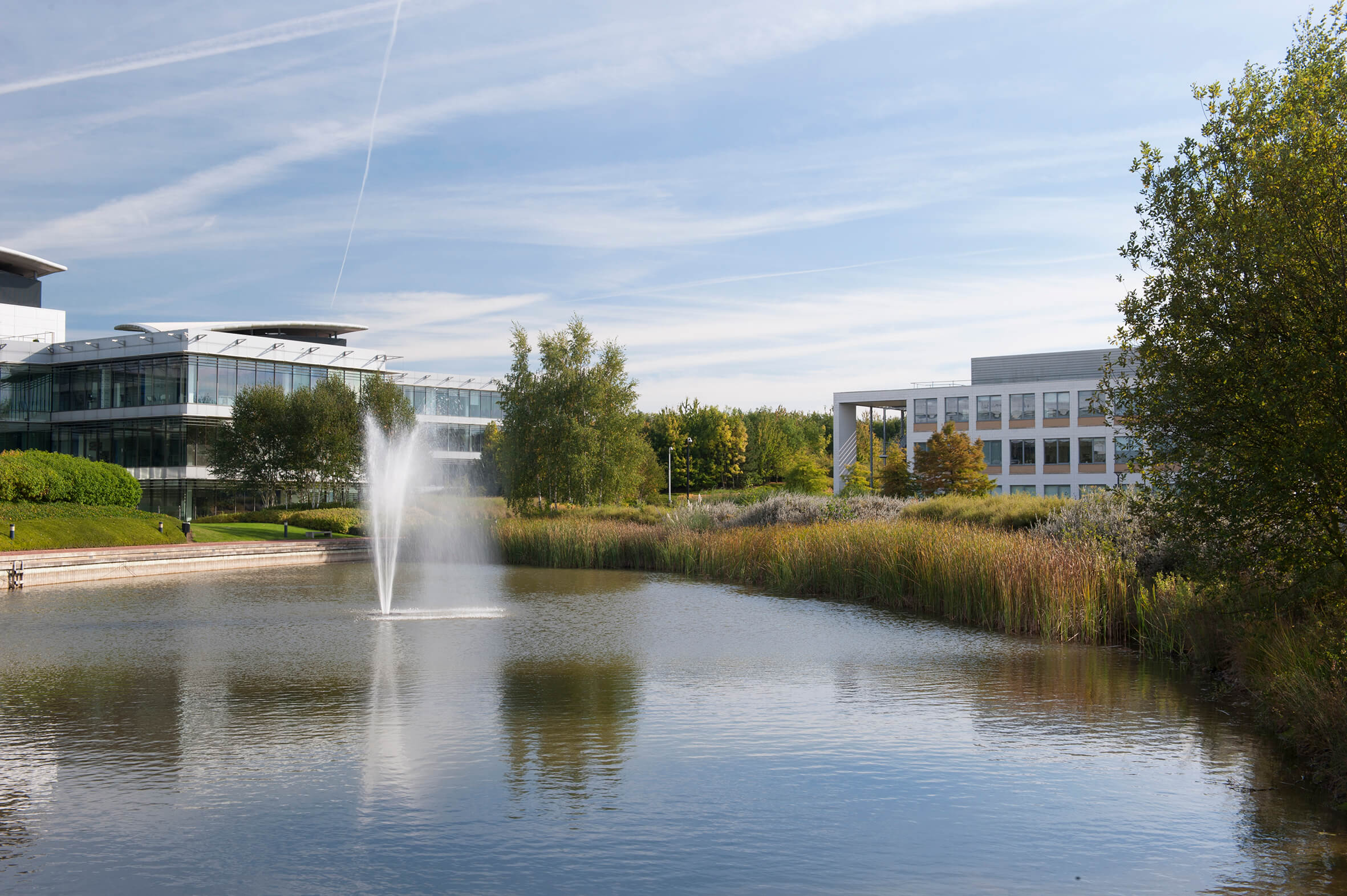
How can we help you?
SFA (Oxford) provides bespoke, independent intelligence on the strategic metal markets, specifically tailored to your needs. To find out more about what we can offer you, please contact us.